Nuclear excited states may be key to search for new flavor-violating physics
Prepared by Evan Rule
Collider-based experiments continue to push the energy frontier in search of new particles, including those associated with supersymmetry, leptoquarks, heavy neutrinos, or a more complicated Higgs sector. At the same time, important constraints on new physics also come from precision, low-energy experiments that test predictions of the standard model. Flavor violation among the neutrinos, discovered in solar and atmospheric neutrino experiments, indicates that lepton flavor is not an exact symmetry of nature. If similar flavor violation were to be observed among the charged leptons (electron, muon, and tauon), it would be definitive evidence of new physics beyond the standard model.
One of the most tightly constrained charged-lepton-flavor violation (CLFV) processes is conversion. In this process, a muon is first captured onto an atomic nucleus, and we look for a final state containing only an electron and no neutrinos. A new generation of experiments, Mu2e at Fermilab and COMET at Japan’s J-PARC, are aiming to improve the experimental sensitivity on
conversion by a factor of 10,000 by the end of the decade, probing energy scales as high as 10,000 TeV. Over their respective lifetimes, Mu2e and COMET will each capture roughly
muons onto targets composed of
Al nuclei, hoping to detect just a handful of
conversion events.
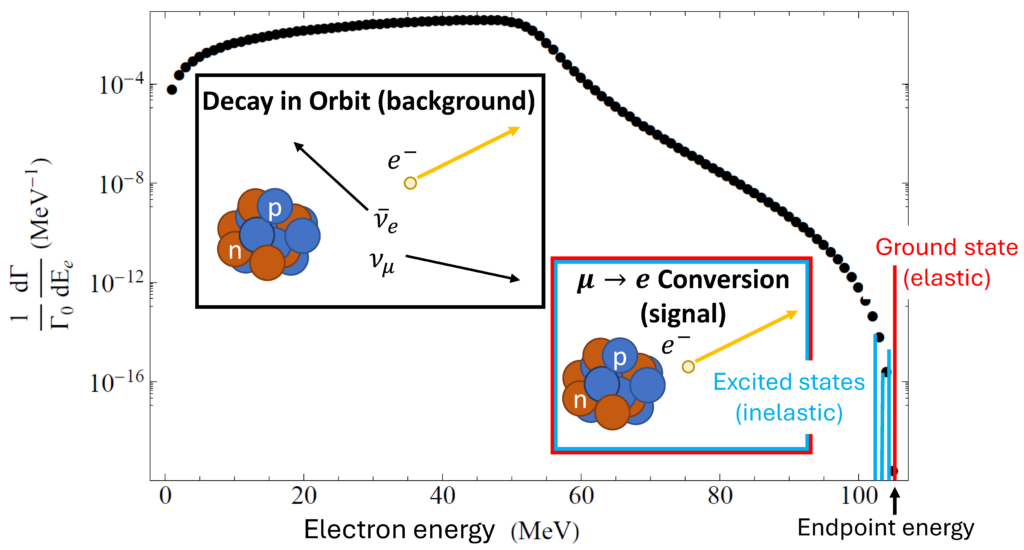


In conversion experiments, the captured muon has a precisely known energy. If it were to convert to an electron and zero neutrinos (without exciting the nucleus), then the resulting electron would have exactly the energy of the initial bound muon, called the endpoint energy. To say with certainty that CLFV occurred, experimentalists must be able to distinguish electrons produced via conversion from electrons originating in the standard-model decay
, which is known as decay-in-orbit (DIO). Because the two neutrinos always carry away some amount of energy, background DIO electrons must have energies below the endpoint energy, as illustrated in Figure 1.
Conventionally, experiments focus on the case where the nucleus remains in the ground state throughout the process — which we refer to as elastic conversion — since this is the region where the DIO background should be (essentially) zero. In previous work, we identified 12 independent CLFV operators that could mediate elastic conversion. Typically, experimentalists are interested in constraining a single number, the total rate for elastic
conversion. In this sense, a positive detection of CLFV at Mu2e and/or COMET does not provide any information about the operator responsible: One can dial the coefficient of any candidate operator to reproduce this single measured value. To learn more about the CLFV physics — for example, does it couple primarily to nuclear spin or to nuclear charge — one would need to repeat the experiment with many different nuclear targets, a potentially costly and time-consuming task.
The Mu2e and COMET experiments will measure the spectrum of electrons near the endpoint energy, not just the elastic rate. What new information about the nature of CLFV will this spectrum provide? A large amount of energy is available as the muon decays into an electron, and just a small fraction of this energy can allow the nucleus to transition to an internal excited state. Any energy used to excite the nucleus is subtracted from the energy of the resulting signal electron, and as one moves away from the endpoint energy, signal electrons must compete with an increasing DIO background. Still, if the energy of the nuclear excited state is not too large, then the resulting signal electron is only slightly below the endpoint energy, where the DIO background should still be low. Al, the target of the Mu2e and COMET experiments, has three relatively low-lying excited states with energies
MeV.
Theoretically, the elastic conversion process produces a mono-energetic electron. In practice, however, the energy is smeared out by experimental effects. Recently, the Mu2e collaboration performed a detailed simulation of their experimental pipeline, leading to the continuous signal shown in the top panel of Figure 2. Beginning from this elastic signal, we estimated the signal from excited state transitions by 1) shifting the momentum of the signal electron by the nuclear excitation energy and 2) rescaling the amplitude of the signal depending on the relative branching ratio for the excited state transition compared to the ground-state transition. This latter quantity depends on a ratio of nuclear response functions, which we estimate using the nuclear shell model.
Figure 2 compares the expected electron spectrum for Run I of the Mu2e experiment in 3 different CLFV scenarios. In the case of coherent conversion, the ground-state rate is enhanced by nearly 1,000 times compared to the excited-state transitions. As a result, the excited-state transitions have no appreciable effect on the spectrum shown in the top panel. If CLFV is mediated by a spin-dependent operator, then the elastic process is no longer enhanced, and the excited-state contributions produce a significant distortion in the measured electron spectrum relative to the elastic baseline (middle panel). In the case of transverse-spin-mediated conversion, the inelastic contributions are even stronger, creating a distinct second peak in the electron spectrum (bottom panel). Importantly, any observed excess relative to the elastic baseline would rule out coherent conversion as the driving mechanism of CLFV.
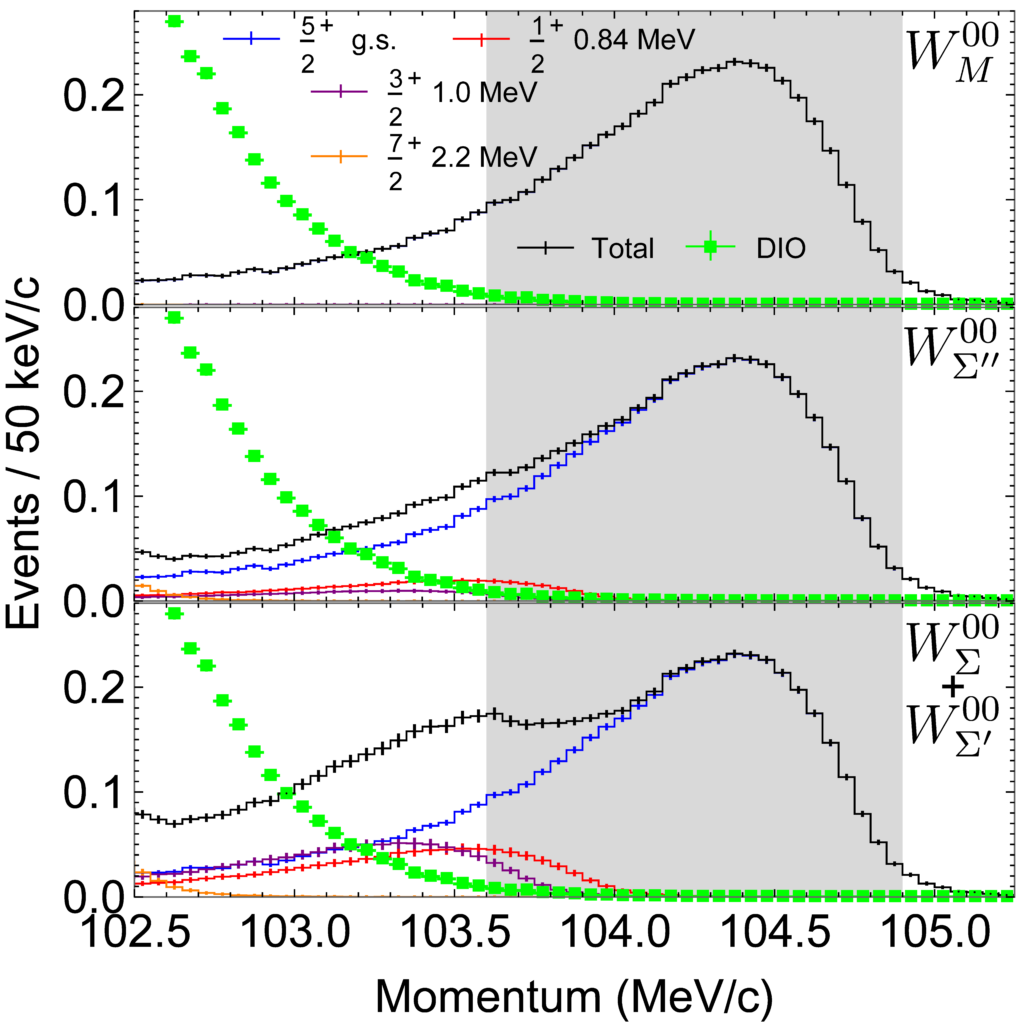


Read More: