Large and massive neutron stars: Implications for the sound speed in dense QCD
Prepared by Sophia Han
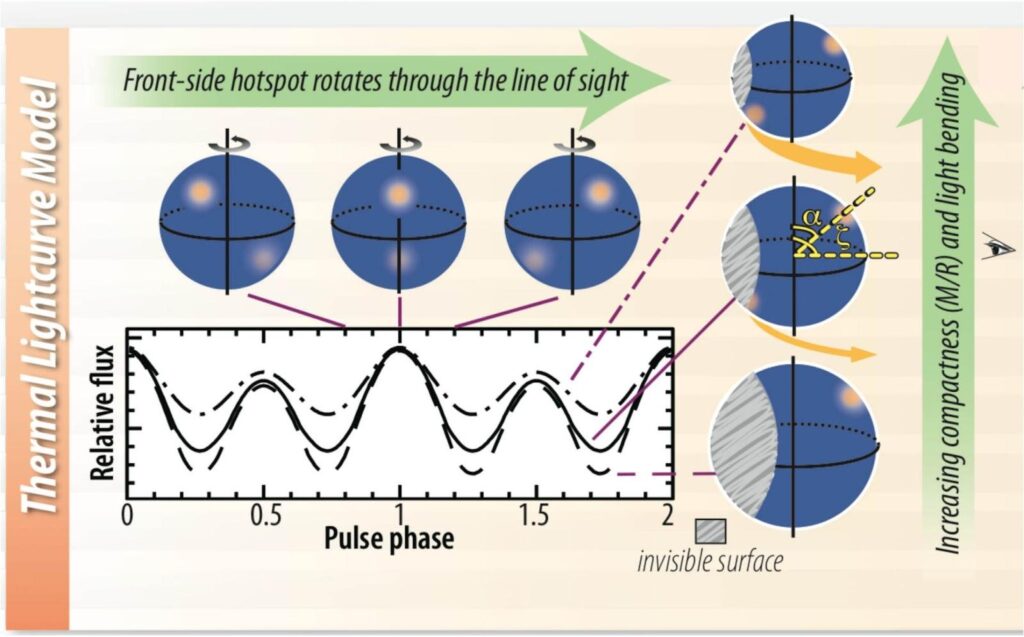
How big are neutron stars? This is a simple yet crucial question, because precise measurements of the size of neutron stars are required for having a solid grasp on the state of the densest material in their interiors and for differentiating between theoretical models. In particular the neutron-star equation of state (EOS) which relates internal pressure to energy density, and its derivative, the squared speed of sound cs2, can be constrained significantly depending on the resolution of radius measurements. The first detection by the LIGO-Virgo Scientific Collaboration of gravitational wave emission from a binary neutron star merger, GW170817, marked a breakthrough as a true multi-messenger event, and the measured distortion of the two component stars due to tidal forces before their final collision favors models that predict small radii <13.6 km for canonical-mass neutron stars around 1.4 times the mass of our Sun. The preference for small neutron stars in this mass range indicates that matter at intermediate densities cannot be “too stiff”, or equivalently the pressure should not be rising with density too rapidly. This is well in line with state-of-the-art microscopic calculations of nuclear matter, which suggest a relatively low speed of sound in the outer core region of neutron stars.
More recently, it has been revealed that we obtained unprecedented knowledge on the size of very massive neutron stars about twice the solar mass M⊙–possibly in the vicinity of a theoretical limit above which the neutron star cannot help collapsing into a black hole. This was made possible by NASA’s Neutron star Interior Composition ExploreR (NICER) telescope launched and stationed on the International Space Station in 2017, dedicated to collect and closely track x-ray emission from “hotspots” on the surface of fast-spinning pulsars. The latest analyses of NICER data on the most massive known neutron star, PSR J0740+6620 with its mass precisely determined to be 2.08+/-0.07 M⊙ through pulsar timing, were performed independently by two groups, and the results released point to a range of 11.4–16.1 km for its radius at the 68% credibility level. Despite the still large uncertainty, NICER’s measurements for the first time implies that radii of massive neutron stars (such as PSR J0740+6620) could be large, and allows the possibility of a scenario where the size of massive neutron stars is greater than that of canonical-mass neutron stars. For standard EOS models where the sound speed increases gradually with the density, these conditions are, however, not easy to be realized.
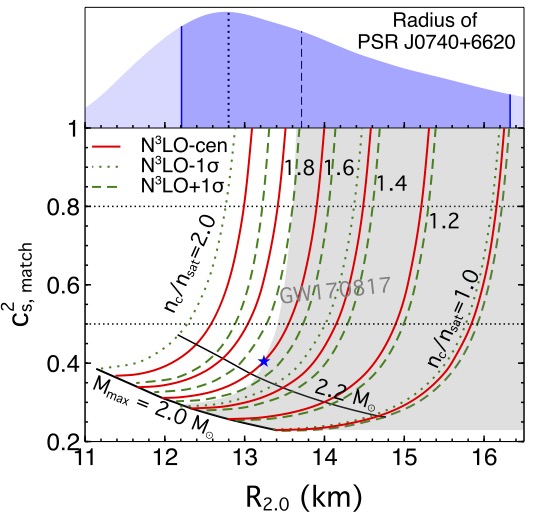
Top panel: The joint probability distribution (on an arbitrary scale) for the radius of PSR J0740+6620 as inferred from NICER and XMM-Newton data. Bottom panel: minimally required values of the core sound speed (y-axis) vs. inferred radii for a two-solar-mass neutron star (x-axis). Red solid curves refer to results based on χEFT calculations of the nuclear EOS up to next-to-next-to-next-to-leading order (N3LO), for matter at densities below n_c; green dashed and dotted curves represent ± 1σ uncertainties. Lower bounds on the neutron star maximum mass are indicated by the solid black lines. The gray region is excluded by the tidal deformability measurement of GW170817.
In this work, we study the implications of large massive neutron stars for the sound speed in their high-density inner cores, utilizing recent chiral effective field theory (χEFT) calculations of the nuclear EOS with quantified theoretical uncertainties at moderate densities encountered in the outer core. We find that a remarkably high sound speed surpassing 75% (66%) of the speed of light is required in PSR J0740+6620’s inner core at the 68% (95%) credibility level, if radii >13 km are realistic and confirmed in the future by improved measurements and analyses. This is a direct consequence of the modest pressures at 1-2 times the nuclear densities predicted from theory and also favored by the small deformations of canonical-mass neutron stars inferred in GW170817. The steep increase in the sound speed hints at a rapid transition from soft-ish neutron-rich matter to some form of very strongly interacting matter that is substantially stiffer, of which the nature remains unknown. Mapping out the behavior of EOS and the sound speed profiles in this window will improve our understanding of fundamental theories such as quantum chromodynamics (QCD) at high densities and low temperatures; towards this goal coherent effort and significant advances in observations, microscopic calculations, as well as terrestrial experiments are expected in the next 5 to 10 years.
Header image: Choreography of NASA’s Neutron star Interior Composition ExploreR (NICER) as it studies pulsars and other X-ray sources from its perch aboard the International Space Station. (credit: NASA’s Goddard Space Flight Center)
Read More:
NASA’s NICER Probes the Squeezability of Neutron Stars (NASA.gov)